
The next step after ITER will be a demonstration power plant - or DEMO - that will demonstrate the viability of producing large-scale electricity from fusion.
Fusion is one of nature's most spectacular achievements. Billions and billions of fusion furnaces, the Sun among them, are flaring in the Universe, creating light and energy.
Some seventy years ago scientists understood the physics behind this wonder: the Sun and stars transmute matter, patiently and tirelessly transforming Hydrogen nuclei into Helium atoms and releasing huge amounts of energy in the process.
With this knowledge came the ambition to reproduce, here on Earth, what was happening in the innumerable stars of the Universe. But harnessing the energy of the stars was to prove a formidable task, more complex and arduous than anticipated.
20th Century Fusion

The world's first tokamak device: the Russian T1 Tokamak at the Kurchatov Institute in Moscow. It was the first device to use a stainless steel liner within a copper vacuum chamber.
A major breakthrough occurred in 1968 in the Soviet Union. Researchers there were able to achieve temperature levels and plasma confinement times - two of the main criteria to achieving fusion - that had never been attained before. The Soviet machine was a doughnut-shaped magnetic confinement device called atokamak.
From this time on, the tokamak was to become the dominant concept in fusion research, and tokamak devices multiplied across the globe.
Producing fusion energy, it soon became clear, would require marshalling the creative forces, technological skills, and financial resources of the international community. The Joint European Torus (JET) in Culham, U.K., in operation since 1983, was a first step in this direction. JET is collectively used by the EURATOM (European Atomic Energy Community) Associations from more than 20 European countries. In 1991, the JET tokamak achieved the world's first controlled release of fusion power.

Research and development for ITER pointed to coconut-shell charcoal as the most efficient coating material for the cryopanels that keep the vacuum in the ITER Tokamak clean. A supply of 2002 Indonesian coconut charcoal is being stored at the Karlsruhe Institute of Technology in Germany for ITER's cryopumps. Photo: Peter Ginter
Achievements like these have led fusion science to an exciting threshold: the long sought-after plasma energy breakeven point. Breakeven describes the moment when plasmas in a fusion device release at least as much energy as is required to produce them. Plasma energy breakeven has never been achieved: the current record for energy release is held by JET, which succeeded in generating 70% of input power. Scientists have now designed the next-step device - ITER - which will produce more power than it consumes: for 50 MW of input power, 500 MW of output power will be produced.
ITER will begin writing the chapter on 21st century fusion.
Plasma Confinement [SIZE]
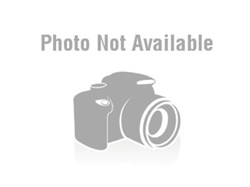
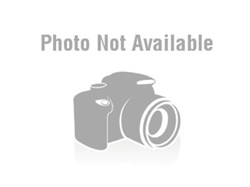
Magnetically confined plasma in the Korean superconducting tokamak, KSTAR. The extreme temperature plasma radiates in a spectrum that our eyes can not see. What is visible in this image are the colder regions on the outer edge of the plasma. Photo: KSTAR.
Physicists have been exploring the properties of plasmas within tokamak devices since the 1960s. The doughnut-shaped torus of the tokamak represented a major break-through in plasma science at the time: here temperature levels and plasma confinement times reached levels that had never been attained before.
The ITER Tokamak chamber will be twice as large as any previous tokamak, with a plasma volume of 830 cubic meters. Left to itself, the plasma would occupy all of the space in the chamber, however no material could withstand contact with the extreme-temperature plasma. Scientists are able to contain or 'confine' the plasma away from the walls by exploiting certain of its physical properties.
Plasmas consist of charged particles - positive nuclei and negative electrons - that can be shaped and confined by magnetic forces. Like iron filings in the presence of a magnet, particles in the plasma will follow magnetic field lines. The magnetic field acts as a recipient that is not affected by heat like an ordinary solid container.
In ITER, different types of magnetic fields will work in subtle combination to shape the plasma into the form of a ring - or torus - and isolate the very hot plasma from the relatively cold vessel walls in order to retain the energy for as long as possible. The vacuum vessel is the first safety confinement barrier, and will not be in contact with the plasma.
In my opinion,the time has come for Malaysia to build it's own nuclear power plant since we already have high chances to make it..
ReplyDeleteYOGESWERAROW A\L SREE RAMALU
ME084029
yoges.sphinx_cruisevirgo88@yahoo.com
thanks for givinv brilliant opinion.
ReplyDeletehope so.and for sure that the time will come...2025 or newer.
Plasma Confinemen,inside the chamber what will be the extreme-temperature for the plasma?
ReplyDeletekumaran
kmrn_siva@yahoo.com
As stated above,plasmas can be shaped and confined by magnetic forces.
ReplyDeleteIs there any different effect regarding the different shapes and confined produce?
name: Mohamad `Adif Bin Jemarin
email: ucukadif@yahoo.com
hye,
ReplyDeletewow..the topic progress in fusion is really interesting.izit the picture in that topic are applied in our country which mean that will demonstrate the viability of producing large-scale electricity from fusion.
Sathis Kumar Kanesan
sinkansen_19@yahoo.com